What about the waste?
Rather than a problem, nuclear waste could be the catalyst for a fossil-free future
Over the past several weeks I have written nearly 6,000 words on nuclear energy, mostly casting it in a positive light, while avoiding the topic of waste altogether. This might seem like a suspicious omission, considering that waste is the primary concern associated with nuclear power. In my experience, people tend to fear the dangers posed by radioactive waste more than the potential for meltdowns like those at Chernobyl and Fukushima. How, then, can one advocate for nuclear power while sidestepping the issue of waste?
Fear not, dear reader, for you have come to the right place today. In this final installment of my series on nuclear power, I will explore and explain nuclear waste in detail: what it is, its longevity, current methods for managing it, and future disposal plans. Furthermore, I will compare nuclear waste to alternative energy sources, and I will make the argument that it holds the key to an energy transition that can decouple human progress from consumption of fossil fuels.
I recognize the visceral fear that radioactive waste can evoke in many people. I used to count myself among that group. However, today I believe my concerns were largely misplaced, stemming from a dearth of accessible and factual information on this topic.
Before we proceed, I’d like to draw your attention to the single best video out there about nuclear waste. In fact, if a half hour of your time is all you can spare for this subject, I urge you to stop reading right now and watch the video instead. In these twenty-nine captivating minutes you’ll discover the slowest measurable speed, witness a train crash at 160 km/h, enjoy not one but two movie reviews, explore an art gallery adorned with radioactive materials, and encounter phrases such as “Jesus hole” and “liger box.” Trust me, it will all make sense, and I promise it will be worth your time.
Go on, click that play button.
Alright, you’re still with me. Let’s go then.
All energy production inevitably generates waste
In my opinion, the pointed question of “what about the waste?” is precisely the right mindset to have. In fact, we don’t hear it being raised often enough when discussing energy production and industrial activities in general. After all, it was our indifference toward fossil fuel waste that led us into the climate change predicament we find ourselves in. Since 1750, humanity has been emitting 18,000,000 tons of CO2 into the environment every single day. And that’s just the average of the past 270 years; today, the figure surpasses 100,000,000 tons of daily CO2 waste, not to mention the methane and other greenhouse gases. Only recently, after facing civilizational peril under the threat of climate change, have we decided to do something about all that waste.
In the realm of energy, there are no perfect solutions; there are only trade-offs. Every energy source comes with its drawbacks, most notably concerning waste.
Following is a short summary of the waste produced by low-carbon electricity sources. I want to emphasize that I strongly advocate a massive expansion of all these power sources. They are the foundation of a new global energy system that is absolutely crucial for effectively combating climate change. However, we can’t ignore the detrimental impacts associated with these energy sources simply because emit fewer greenhouse gases. Each type of waste is different, the amount produced varies greatly, and some are more challenging to address than others. But to ensure a truly sustainable energy system, we need a plan for managing the waste produced by all these sources. Negative externalities have already caused enough problems for human civilization; let’s not allow history to repeat itself.
Wind Waste
Wind turbines are colossal structures designed to endure the elements and withstand immense forces, making them virtually indestructible. Their blades, made of plastic composites, not only require a lot of energy to manufacture but are not feasible to recycle because there is nothing of value to recover. Consequently, we are witnessing the early stages of an escalating issue: enormous blades being deposited in specially designated landfills, where they will essentially remain forever. With wind turbines operating for 20-30 years and the increasing rate at which they are being built, the accumulation of waste will become a significant problem this century if left unaddressed.
Solar Waste
Unlike wind turbines, solar panels contain valuable materials that can be reclaimed. Regrettably, recycling is currently minimal because it is not economical, and solar panels are already showing up at landfills. By 2050, an estimated 78,000,000 tons of solar panels will reach the end of their lifespan, making sustainable solar recycling an imperative, considering our reliance on photovoltaic cells for electricity. I am optimistic that a recycling industry will emerge soon, but not all materials will be recovered, and a substantial portion will continue to be disposed of in landfills where they leach out toxic substances such as lead, arsenic, and cadmium. Additionally, solar requires intensive mining and processing of raw materials, activities that generate large amounts of toxic waste and other forms of pollution.
Hydro Waste
Among major energy sources, hydroelectric power generates the least amount of waste, but it is not without its drawbacks. Dams release methylmercury, an extremely hazardous substance that infiltrates the food chain, causing detrimental health effects for all animals including humans, especially indigenous communities residing near dam sites. Hydro dams also emit methane for decades following flooding of an area, as a result of decomposing plant matter. And then there are the well-known adverse environmental impacts of hydro projects, such as reduced river flows and water quality degradation, sometimes leading to the destruction of entire ecosystems.
Geothermal Waste
Geothermal is unlikely to play a major role in energy production anytime soon, but it deserves attention considering the growing interest surrounding it. Some geothermal systems emit a small amount of greenhouse gases, but the latest closed systems do not produce any waste during operation. However, the construction of a geothermal plant is effectively a fracking operation which releases methane during the drilling through shale rock. Although rare, damaging earthquakes triggered by geothermal projects can and do happen.
How about nuclear waste?
Nuclear power is different in that the industry is required to take responsibility for and contain all its waste products. That is mandated by regulators, such as the Nuclear Regulatory Commission in the United States, and overseen by international agencies, such as the International Atomic Energy Agency, due to the extreme danger presented by mishandled radioactive materials.
I believe there’s a crucial aspect that most people tend to overlook in this debate: the nuclear industry offers a framework for safely managing hazardous waste in sustainable industrial practices. It serves as a compelling case study, showcasing successful application of this framework over several decades.
What is nuclear waste?
In common parlance, “nuclear waste” generally refers to used fuel, also known as high-level waste (HLW) which accounts for 95% of the radioactivity but only 3% of the waste volume generated by commercial nuclear plants worldwide. Throughout this article, the term “nuclear waste” is used to specifically refer to HLW. The terms “used fuel” and “spent fuel” are used interchangeably, although I find “used fuel” to be more precise, for reasons that will become evident by the end of this article.
Intermediate-level waste (ILW), constituting 7% by volume, primarily comprises reactor components and materials used in fuel reprocessing. The vast majority of the all the waste falls under the category of low-level waste (LLW), which constitutes 90% by volume but only 1% of the radioactivity. LLW includes things like contaminated tools and clothing and is generated by nuclear facilities, as well as hospitals and research laboratories. Both low- and intermediate-level waste can be safely disposed of near the surface, typically in engineered landfills situated close to power plants. These disposal sites are classified as nuclear facilities and are subject to strict regulations to ensure complete containment of all waste.
However, LLW and ILW, already safely and permanently disposed of and not very hazardous in the long term, pale in comparison to used fuel, the primary focus of fear and debate.
In the history of nuclear power, about 450,000 tons of spent fuel have been generated globally, with another 10,000 tons added to the inventory each year. While that is indeed a large volume, note that it is many orders of magnitude less than the current daily amount of fossil fuel waste. Furthermore, coal ash itself is radioactive and contains other harmful substances such as mercury and arsenic, which are currently being released into the environment unchecked and will remain extremely hazardous forever. HLW is certainly in a class of its own, but unlike waste products from other industries, 100% of it is securely contained and accounted for.
From reactor to storage
Despite The Simpsons having eternalized nuclear waste in the collective imagination as a slimy green goo leaking from rusty old barrels, that image bears no resemblance to the actual thing.
Nuclear fuel consists of ceramic pellets of enriched uranium, each about the size of a fingertip. Before they are assembled by the hundreds to be inserted into the reactor, the pellets are inert and can be handled without protective gear. Inside the reactor core, the pellets are bombarded by neutrons, producing heat energy that is then channeled to a steam generator to make electricity. After 18-36 months, when the chain reaction becomes less efficient, the fuel is removed from the reactor by a robotic arm and immediately transferred to a cooling pool.
The cooling pool is exactly what it sounds like: a big pool of clear water that cools the fuel while allowing it to shed most of its radiation. Water serves as an effective shield, with radiation levels outside the pool barely surpassing background levels, enabling plant workers to move around the pool without protective clothing. In fact, radiation inside the spent fuel pool is lower than outside, as water blocks even the natural cosmic radiation that constantly bombards the Earth’s surface.
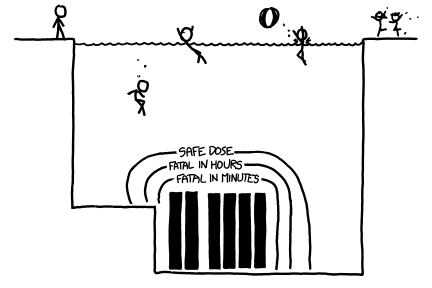
Since the fuel in the pool continues to emit a lot of heat, continuous pumping of cool water is needed. This is one of the reasons why nuclear plants are equipped with multiple backup systems in the event of a power failure.
After several years at the bottom of the pool (usually from 5 to 20, varying by facility) the ceramic fuel pellets have sufficiently cooled to be moved to dry storage.
The used fuel is then placed in concrete boxes and stored within dry casks. These casks are steel canisters with walls half a meter thick and can be stored in warehouses or open-air areas adjacent to the plant. The dry casks effectively shield all the radiation emitted by the fuel and are basically indestructible. The various attempts to compromise the integrity of dry casks are great fun to watch, my favorite being a missile strike:
Dry casks are designed to securely contain the used fuel for approximately 100 years. Beyond this point, a permanent disposal solution is needed. Currently, such a solution is not yet fully available, but extensive plans and ongoing projects are in place (read on for details.)
How long is the stuff radioactive for?
That question has been a longstanding concern, and the answer can be somewhat misleading: the truth is that nuclear waste remains radioactive forever. But so does everything else. Radiation is all around us in the background and even in the food we eat, especially if you like bananas or Brazil nuts. Bananas, for example, contain potassium-40, a naturally occurring radioactive isotope. I am not suggesting that the banana bread in your Sunday brunch is as harmful as a spoonful of plutonium-239. Instead, it is more useful to ask how radioactive something is at any point in time compared to established safe levels.
Enter the BED, or banana equivalent dose (note: Sv = sieverts, the international unit for measuring ambient radiation. I don’t understand it either, it’s fine to just stick with banana equivalents)
The radiation exposure from consuming a banana is approximately 1% of the average daily exposure to radiation, which is 100 banana equivalent doses (BED). The maximum permitted radiation leakage for a nuclear power plant is equivalent to 2,500 BED (250 μSv) per year, while a chest CT scan delivers 70,000 BED (7 mSv). An acute lethal dose of radiation is approximately 35,000,000 BED (3.5 Sv)
Yes, eating 35 million bananas in one sitting will kill you from radiation poisoning (please don’t try this at home.) Do check out the Wikipedia above, especially the useful chart that puts in perspective the radiation doses from several sources, including from living near a nuclear plant, compared to maximum allowed levels.
Back to the waste, after 40 years, 99% of its radioactivity will be gone, but the 1% that remains can still be as harmful as consuming millions of bananas (due to the radiation, if not the indigestion.) After 600 to 1,000 years, there will be no more gamma radiation, which is the type that can cause damage from a distance. At this point, holding spent fuel with bare hands would not pose a major risk, although you still wouldn’t want to eat it. And because radioactive decay is exponential, meaning a rapid initial decline followed by a slow decay, it could take around 200,000 years for nuclear waste to reach natural levels of radiation. But that does not mean that it remains hazardous for hundreds of thousands, or even thousands of years. Nevertheless, one thousand years is still an incredibly long time, and for practical purposes we should consider nuclear waste as potentially hazardous indefinitely.
Long-term plans for nuclear waste
Deep Geological Repository
The scientific consensus for the permanent disposal of nuclear waste has long been to bury it deep underground. Though the word ‘bury’ doesn’t quite convey the right concept, it’s like saying that I am ‘flying’ whenever I jump a couple of feet in the air.
A Deep Geological Repository (DGR) is a network of tunnels built up to a kilometer deep underground in impermeable bedrock that has remained stable for millions of years. The engineering details are extensive (learn more here), but it suffices to say that DGRs employ a combination of engineered and natural barriers to isolate nuclear waste from the environment far beyond the detectable presence of radioactivity above natural levels.
Finland is set to start operating the world’s first DGR in 2024, with several other countries following suit in the coming years. However, a handful of national projects will not be sufficient to dispose of the global inventory. Since 2004, the International Atomic Energy Agency has been calling for multinational DGRs capable of handling nuclear waste from any country.
The private sector has embraced this vision, and plans for multinational DGRs are already being put into action (disclaimer: I recently advised a company working on such a solution.) Another promising approach is to use boreholes to bury small canisters of used fuel at even greater depths, eliminating the need for extensive excavation projects.
Reprocessing
Although modern reactors generate immense energy from small amounts of nuclear fuel, they only convert a tiny fraction of the fuel mass into energy. Spent fuel still contains fissile uranium-235 which can be extracted through reprocessing to produce more fuel.
Yes, nuclear waste is recyclable. However, reprocessing is not economically viable, so it is seldom carried out. It is also fraught with challenges as it involves separating elements within the spent fuel, a small portion of which is fissile plutonium. Plutonium can be used in certain types of reactors, but it is also one of the components in the production of atomic weapons.
France is the leader in used fuel reprocessing today, recycling not only their own used fuel but also that of other countries. As a result, France has a relatively small nuclear waste inventory despite boasting one of the world’s largest nuclear fleets.
Reactors that consume spent fuel
High-level nuclear waste still contains about 90% of its potential energy, so the fuel can hardly be considered ‘spent’. If extracted, this clean energy has the potential to power civilization for centuries, and the resulting radioactive material would be hazardous for significantly less time. While we are not yet capable of extracting all the potential energy from the used fuel, we do know how to build reactors that can squeeze a lot more juice out of that lemon.
Canada has designed a reactor that can consume uranium found in natural form (i.e., not enriched) as well as used fuel. In a neat nuclear ecosystem, Canadians can supply their CANDU reactors with their ample uranium reserves, as well as with the waste produced by their conventional nuclear plants.
Fast reactors, also called breeder reactors, were developed in the 1950s but have not been widely used commercially since then. They are now gaining popularity with the increasing number of small modular designs. These reactors can generate, or “breed”, their own fissile material from uranium-238 (which constitutes 95% of the content in used fuel), and from thorium. Both elements are more abundant in nature compared to fissile uranium-235, thus significantly reducing the need for mining and enriching.
Despite the high density of nuclear fuel, currently we don't use it very efficiently. It takes 16 tons of mined uranium to produce 1 terawatt-hour of electricity, with only 1% of the total amount of mined uranium undergoing fission. Fast reactors could increase the fissioning rate up to 99% of the mined uranium, making it possible to produce the same 1 terawatt-hour of electricity using just 0.1 tons of mined uranium. This would enable us to repurpose existing nuclear waste stockpiles as fuel and greatly reduce the need for fresh mining. The resulting waste would also be significantly less radioactive, since the long-lived materials are consumed in the fast reactors, and the remaining isotopes would decay after 200-300 years.
It might not be waste after all
There is no question that high-level waste is extremely dangerous for an extended period of time. But we know how to make it safe. Remarkably, in the history of commercial nuclear power there has not been a single recorded death caused by nuclear waste. In stark contrast, the death toll from fossil fuel waste is estimated in the millions per year.
Nuclear power stands out for generating less waste than nearly any other source of energy, especially among flexible sources. Furthermore, the small volume of waste is meticulously isolated, and managed more securely than any other hazardous material on the planet.
Thinking of used fuel solely as “waste” overlooks its potential as a carbon-free energy source. Where some see only a dangerous substance to be feared, others see the opportunity to meet the world’s clean energy needs far into the future. As environmental group RePlanet points out in their What A Waste report, if used efficiently, nuclear fuel
is essentially limitless: sufficient to supply a growing human civilisation with carbon-free energy for tens of thousands of years, and likely far longer.
Don’t let these go to waste
This short Twitter thread makes the point that there are extremely dangerous things that we engage in routinely because we’ve made them safe, like flying thin, pressurized metal tubes through the air at 900km/h at altitudes of 30,000 feet over vast oceans.
This video is shorter than the one I recommended at the top and it goes a bit deeper into the uranium fuel cycle and reprocessing.
Wikipedia’s Nuclear fuel cycle is the best accessible yet detailed explanation I found of differences between each type of reactor and fuel.
Not waste related, but I can’t finish this nuclear series without linking to this fascinating account of how stray dogs left behind in the Chernobyl evacuation gave rise to a population of wild dogs living within the exclusion zone.